
Introduction to Sodium-Ion Battery Technology
Starting in 2015, prices for lithium almost tripled to more than $20,000 a tonne in just ten months. The metal is the central component of lithium-ion batteries, used to power the majority of the gadgetry that Western society now takes for granted, everything from laptops and smartphones to electric vehicles, and has become indispensible.
Demand for the metal is insatiable. Bloomberg reports that electric car production is set to increase more than thirtyfold by 2030, and the race is on in laboratories from California to New South Wales to develop a high-efficiency, low-cost alternative to the now ubiquitous lithium-ion batteries, which are less environmentally damaging than those containing heavy metals such as cadmium and mercury.
Could sodium (Na), lithium’s neighbour in the periodic table, provide an answer? Postdoctoral scholar Min Ah Lee and her research colleagues at Stanford University in the US certainly think so.
“The main challenges in developing a battery that’s more cost-effective than lithium is the absence of high-performing electrode materials,” Lee explains. “There have been demonstrations of sodium-ion batteries that use layered transition-metal oxides and polyanions, which are the sodium versions of the compounds used as lithium-ion battery electrodes.
“However, the layered metal oxides have shown limited cycling stability and air sensitivity, and the polyanions only delivered a moderate energy density, mainly because of the bigger size of Na cation compared with Li cation. Further research is required to get better sodium host materials with higher energy and better stability.”
The sodium-ion battery explained
The prototype developed by the team at Stanford contains a sodium-based cathode, the pole of the battery that stores electrons. The battery’s internal chemistry shuttles these electrons toward a negative anode, in this case made up of phosphorous. The more efficient this process is, the better the battery works.
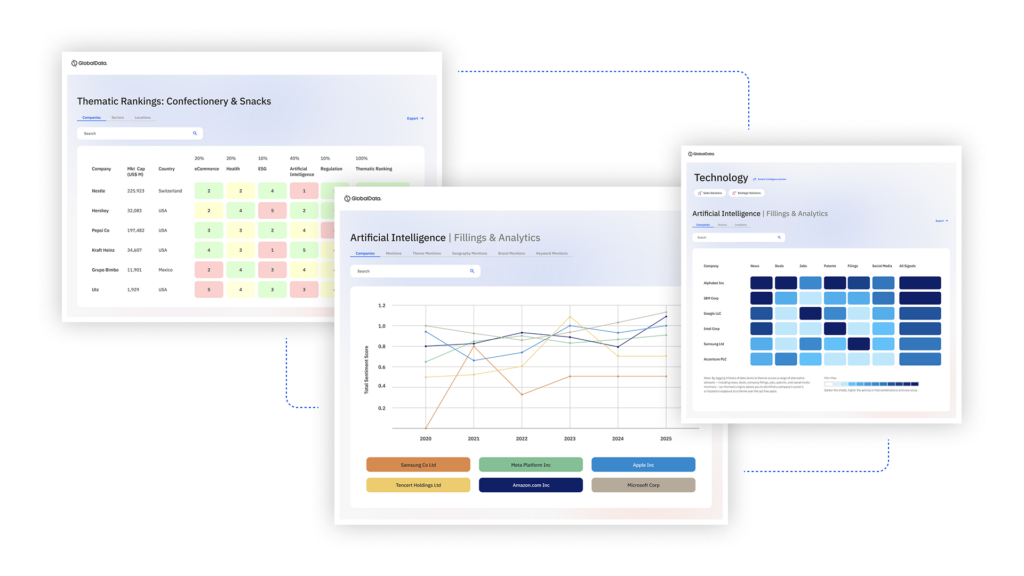
US Tariffs are shifting - will you react or anticipate?
Don’t let policy changes catch you off guard. Stay proactive with real-time data and expert analysis.
By GlobalDataThe team focused on how sodium and myo-inositol – a widely available organic compound found in baby formula and derived from rice bran or from a liquid byproduct of the process used to mill corn – could be used to improve the electron flow, significantly boosting the performance of the battery.
“Organic compounds obtained from natural biomass with minimum energy consumption are an attractive low-cost and sustainable choice for battery electrode materials, provided a high energy density and long cycling stability can be obtained,” says Lee. “Our new cathode comprised of carbon, oxygen and sodium can exhibit a comparable energy density to that of conventional lithium cathode, providing a way to build a cost-effective Na-ion battery for the grid-scale application.”
Myo-inositol costs $150 a tonne compared with $15,000 to mine and refine lithium, and has the potential to create a sodium-ion battery with the same storage capacity that is 80% cheaper.
“We can compare the cathode costs to exhibit the same full-cell energy density for a graphite-lithium nickel manganese cobalt oxide (NMC) lithium-ion battery and our phosphorous-sodium rhodizonate sodium-ion battery,” says Lee.
“The material costs are $30/kg for NMC, and $10/kg for our sodium salt, so the cost per kW/h for NMC in the lithium cell is around $48/kWh, and for our material in the sodium cell is $35/kWh.
“With further development of a better anode having lower operating potential in the future, the cost should be decreased by $20/kWh, with an increase in full cell energy density. We are expecting the wholesale price or cost for mass production of our cathode material to be even cheaper than $10/kg, as it is originated from abundant biomass; for example, corn liquor.”
Making sodium batteries commercially viable
Researchers working on sodium-ion batteries consistently run into the same problem: the lack of a high-capacity anode. Lithium-ion batteries use graphite but this doesn’t work for sodium ions.
“The entire shift from Li to sodium battery is still limited by the performance of anode,” confirms Lee. “Currently, our full-cell energy density is limited by the anode having a relatively high operating potential and limited rate capability.
“We are currently working on developing better sodium anodes to build an ideal full-cell system – which is the real product for the market – so that we can really have comparable full cell energy density in the near future. The safety of sodium battery is also another concern limiting this technology to be viable so research effort should be made in that respect as well.”
How long could it take for a cheaper alternative to become viable and available to the wider market?
“The mass production of our cathode materials and the resource myo-inositol is already available,” says Lee. “Myo-inositol is naturally present in the human body, being a cell membrane’s component, and is found in many foods, particularly corns, nuts, fruits. Inositol is used as a nutritive supplement in infant formula and is available as an over-the-counter nutritional supplement.
“The synthesis sodium rhodizonate from myo-inositol to is through oxidation in aqueous solution at room temperature, which should be scalable.”
The team also optimised the battery’s charge-recharge cycle to enable it to efficiently store and deliver electricity from, for example, a rooftop solar array so it can run house lights at night.
Charged up: alternatives to sodium-ion solutions
Sodium-ion battery solutions continue to gain traction worldwide. In France, the National Center for Scientific Research (CNRS) has created a company, Tiamat, with the aim of developing and bringing to market a sodium rechargeable battery by 2020.
In the US, start-up Aquion is developing high-capacity saltwater batteries for energy storage, and researchers at Washington State University are working on graphene-based sodium–ion batteries, while scientists from the University of Wollongong in Australia have developed battery cells based on sodium–ion technology.
Sodium-ion solutions are not the only potential rivals to lithium-ion batteries, however. In March, scientists at EPFL and GRT Group announced that they had built the world’s first integrated power supply unit that can produce electricity from formic acid, using a cost-efficient, sustainable fuel cell.
Using hydrogen to produce electricity produces no carbon or particle emissions; however, it has a low energy content by volume, making it difficult to store and transport in its natural gaseous form.
The alternative solution is to use a hydrogen carrier such as formic acid, a litre of which can carry 590L of hydrogen, which forms the basis of the new, integrated formic acid-hydrogen fuel cell.
Another possible solution uses lithium, but this time combined with sulphur (S), as Lee explains.
“One promising advanced lithium batteries system with low materials cost is the Li-S battery,” she says. “But they require complicated nanostructuring processes to make it work, which would add significant manufacturing cost.”
With lithium expensive to mine and refine, the race to find an alternative such as sodium for use in the high-efficiency, low-cost batteries of the future looks set to become even more competitive.