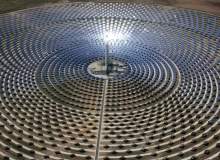
As researchers and renewable energy companies continue to develop solar energy as a technically reliable and economically practical form of power generation, arguably the biggest problem is also the most obvious. The sun transmits more energy to the earth in a single hour than is currently consumed by humans in an entire year, but a host of obstacles and inefficiencies makes reaching even a fraction of this potential a distant dream.
How well do you really know your competitors?
Access the most comprehensive Company Profiles on the market, powered by GlobalData. Save hours of research. Gain competitive edge.
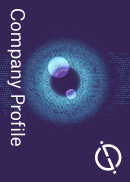
Thank you!
Your download email will arrive shortly
Not ready to buy yet? Download a free sample
We are confident about the unique quality of our Company Profiles. However, we want you to make the most beneficial decision for your business, so we offer a free sample that you can download by submitting the below form
By GlobalDataThe foremost of these obstacles is self-evident – during heavy cloud cover or at night, when energy demand reaches its peak, output from solar arrays drops to zero. It’s this intermittency, not to mention the inefficiency of photovoltaic (PV) solar cells, that keeps solar energy expensive and pushes the majority of renewable investment towards cheaper alternatives like wind.
Modern advances in the field of solar thermal energy and concentrated solar power (CSP) have now offered a number of solutions to the problem of solar intermittency, some of which are now operational. These solutions involve various means of storing solar energy during the day, which can then be accessed at night to maintain consistent, round-the-clock generation.
Through a number of promising technologies, large solar thermal installations can achieve the status of baseload (or 24-hour) electricity generators. Indeed, in the case of Gemasolar in Spain (the world leader in solar thermal), they already have.
The encouraging economics of solar thermal energy storage has pushed solar thermal to the forefront of medium and large-scale solar power generation, despite the tumbling price of PV cells. Two solar energy storage methods, one more developed than the other, have been singled out as particularly promising glimpses at the future of solar power.
Molten salt energy storage
Given its importance to the viability of solar power, storage has been an area of research for some time. A great deal of work has gone into developing battery storage for photovoltaics, but the expense and inefficiency of batteries makes this option impractical for large-scale operations.
But solar thermal power plants harness the sun’s energy to produce heat, which is significantly easier to store efficiently. Nevertheless, substances need to be found to store heat – at the extremely high temperatures found at solar plants – and transfer it back into the power generation process when needed.
Salt, with its ready availability, favourable safety profile and formidable heat retention characteristics – molten salt reportedly loses only 7% of the energy it stores – makes an ideal candidate. The world’s first solar thermal plant with molten salt energy storage (MSES) capability is Andasol-1, a parabolic trough solar thermal power plant that started operations in the Spanish province of Granada in November 2008.
The plant’s usual daytime generation works when sunlight hits parabolic trough mirrors that reflect the heat on to tubes filled with oil, which heats up to 400°C before being used to boil water and creating steam to drive a turbine. But the plant has extra capacity to send heated oil to a heat exchanger, where the heat is transferred to molten salt for storage. The heated salt can then be sent back to the heat exchanger to transfer its stored heat back into oil for turbine operation.
Andasol-1’s MSES method allows the plant to generate electricity for an extra seven and a half hours in the night or during cloudy periods – not quite enough for full 24/7 operation, but certainly enough to increase its annual output from 117,000MWh to 178,000MWh, helping to reduce the cost of its power.
Spain reached the milestone of a 24-hour thermosolar plant a few years later, when Torresol Energy’s 19.9MW Gemasolar concentrated solar power plant opened in May 2011. Gemasolar’s own MSES storage capability extends its operating time by 15 hours, allowing ample supply when the sun goes down and demand goes up.
“The ability to store energy when the sun it at its peak and deliver it when the market demand is at its peak changes everything in the power market,” Torresol’s chief infrastructure officer Santiago Arias told Forbes in 2011. “My fuel cost is zero. Natural gas can simply not compete with us.”
The message is certainly getting through on the other side of the Atlantic, with larger-scale solar thermal plants with MSES capability being developed in the US south-west, including the Solana Generating Station in Arizona and SolarReserve’s Crescent Dunes project in Nevada.
Artificial photosynthesis
That natural process of photosynthesis is one of nature’s most ingenious energy-harvesting techniques. For millions of years, plants have been converting water, sunlight and carbon dioxide into chemical energy, with vital oxygen the only byproduct.
Researchers are now taking inspiration from nature to develop various forms of artificial photosynthesis, an innovation that could provide another means to store solar energy for later use. The basic principle behind the method involves using the sun’s energy to split water into its constituent parts, storing hydrogen to be used as a fuel and releasing oxygen into the atmosphere.
But splitting water molecules is no simple task; a catalyst is required to react to the sun’s photons and kickstart the process. “Splitting water is extremely difficult to do,” said Tom Meyer, who is leading a project to develop the technology at the University of North Carolina’s Energy Frontier Research Center.
“You need to take four electrons away from two water molecules, transfer them somewhere else, and make hydrogen, and, once you have done that, keep the hydrogen and oxygen separated. How to design molecules capable of doing that is a really big challenge that we’ve begun to overcome.”
Meyer’s team solved the problem with the use of dye-sensitised photoelectrosynthesis cells (DSPECs), which contain a molecule and a nanoparticle. The molecule is an assembled catalyst that absorbs sunlight and begins the separation of electrons from the water. The nanoparticle, which is coated with titanium dioxide, rapidly carries the electrons away from the water, leaving the freed electrons to produce hydrogen. The hydrogen could be used as fuel for a future wave of hydrogen-powered vehicles, or stored in fuel cells for on-demand usage.
The process, which can use a range of catalytic materials, including dye-sensitised titanium dioxide, manganese and cobalt oxide, creates a molecular approach to energy storage that, if it can be proved to be stable and efficient, could be a true paradigm shift for solar power. Artificial photosynthesis, which according to the UNC team can be implemented using existing technologies, simply needs to be demonstrated outside the laboratory, although this is something that will take years to achieve.
“When you talk about powering a planet with energy stored in batteries, it’s just not practical,” said Meyer earlier in January. “It turns out that the most energy-dense way to store energy is in the chemical bonds of molecules. And that’s what we did – we found an answer through chemistry.”