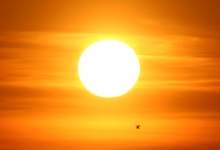
Solar cell efficiency has grown by leaps and bounds since physicist Edmond Becquerel first demonstrated the photovoltaic effect back in 1839 in his father’s laboratory. The first solid state cell, built and demonstrated by Charles Fritts in 1883, registered an efficiency of just 1%; these days, conventional silicon solar cells are achieving efficiency levels of up to 28%. However, both the early inventions and those currently dominating the market are focused on only part of the power. Silicon cells can only convert part of the light spectrum into energy, leaving that which remains invisible to go to waste.
Sean MacDougall, nominee at the Young Professionals Green Energy Awards, and his fellow researchers on the Nanotech project, worked to address this by developing a new method that could take the invisible parts of the light spectrum and make them visible, enabling an increase in efficiency. Here, he discusses the project and what implications it might have on solar efficiency.
Adam Leach: Can you tell us about ‘invisible’ light and its relevance to solar power?
Sean MacDougall: It’s called ‘invisible energy’ because it is invisible to the solar cell. Photovoltaic cells or crystalline silicon cells only absorb light in the visible region. Silicon absorbs up to 1100 nanometres, which is the near infrared, and anything beyond that in longer wavelengths isn’t absorbed and is just transmitted by the solar cell. Crystaline silicon makes up about 85% of the market share and about 20% of the sun’s energy is lost because the photons don’t have enough energy in that region to be absorbed by the photovoltaic cells.
So what we did is place a material behind the solar cell that can absorb the transmitted photons and convert them to visible photons and send them back to the solar cell. It’s basically recycling the transmitted photons.
AL: How did the project come about?
SM: It was part of the EU FP7 initiative and ran from 2010 to 2014 in collaboration with Fraunhoeffer, Phillips and Israeli research institutions. It was put together by Jan Goldschmidt at Franhoeffer and my supervisor Bryce Richards, who was one of the first people to do research into upconversion.
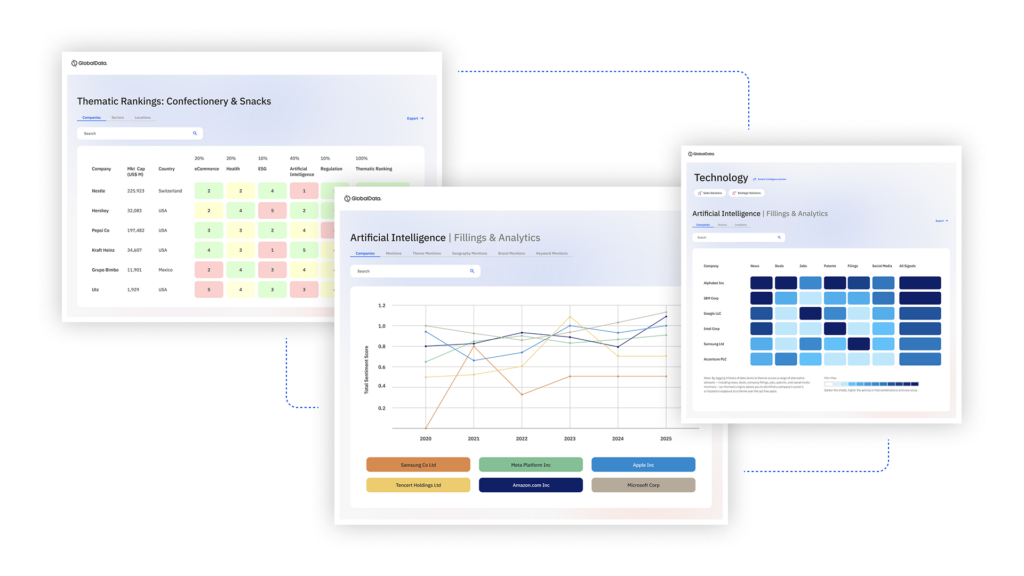
US Tariffs are shifting - will you react or anticipate?
Don’t let policy changes catch you off guard. Stay proactive with real-time data and expert analysis.
By GlobalDataAL: What levels of efficiency did you achieve during the course of the project?
SM: We measured an efficiency of 16.2% which is fairly high considering that the limit is 50% – you have two, at least two, photons transmitted by the solar cell of low energy and you combine them to make a photon of a higher energy which limits the quantum efficiency to 50%. So the 16% we got is in theory 32%.
Researchers in Spain have developed an ‘autonomous’ street lamp with integrated solar and wind turbine technology.
That was fairly close but it was at very high solar concentration, the efficiency is dependant on the power of excitation so you need really high solar concentration to get really high efficiencies. We were finding that you need solar concentrations that were far above what would be achievable just through lenses for the concentration of light.
AL: What levels of efficiency do you think are achievable?
SM: I think the record efficiency for a silicon solar cell is about 28% and that works at 100 suns concentration, which isn’t very high, but we were looking at about 80,000 suns and to achieve that we were looking at advanced technology like quantum dots to reach that level.
There are also stacked solar cells and multi-band gapped solar cells that can achieve about 44% to 45% efficiency that use different semi-conductor materials to absorb different parts of the solar spectrum. They’re all connected in series so each individual part limits the performance of the other one. But with upconversion, there’s no electrical connection to the device; it’s just an optical layer that you can apply to the back of the solar cell, so all the efficiencies are absolute.
That means that even when you’re achieving an efficiency of 16% you can generate significant amounts of current at a low efficiency when using high levels of solar concentration. We were seeing that even with low efficiencies of 16% you could theoretically be generating very high currents that you need for generating power.
AL: What material did you use and what other materials are being investigated?
SM: For the limits of the materials we were getting very close to saturation point. We were using a phosphorescent material and rare earth metal called erbium and for that I would expect a limiting efficiency of 20% overall or a quantum efficiency of 40%. People are now looking at other materials which can do the same thing. We were using inorganic materials but others are looking at organic materials that require much lower solar concentration and things like quantum dots and band gap engineering enables the creation of much better materials.
AL: Tell us about the Shockley Queisser Limit and what implications the invisible light might have on it.
SM: The Shockley Queisser Limit is an equation that basically says that for a single band gap device, such as silicon, the limiting efficiency is 31%. Using the same equations and adding in an upconversion, that could be pushed to something like 48%, so you could significantly improve it. That’s an ideal limit using ideal materials and what we have is very inefficient materials, but at least they do the job. We’re trying to make the best of a bad material, but I think in the future, with the way technology is going, things like quantum dots could be used to achieve that but that might be five to ten years down the line.
AL: How does the performance compare across the different types of solar cells?
SM: One of the biggest problems with using erbium and rare earths is that crystalline silicon has been highly developed and we found that you would actually have to de-engineer it in order to make it work better with upconversion. But there could be potential for it to work with organic solar cells or the thin film cheap products and because they are at such an early stage of development you can integrate the upconversion at the beginning and create a holistic device.
AL: How big an impact do you think upconversion can have on solar power generation?
SM: I think that for crystalline silicon cells, it could have a small impact for high concentration devices so in areas like the Sahara desert or where you have large solar farms.If you can increase the overall efficiency by 1% to 2% that could make a huge deal at large solar farms where you could go beyond the current limit of 31% efficiency, which they’ve almost already reached. It can give them that extra boost and overcome the Shockley Queisser Limit.
For groups that are looking at organic materials – that can work at much lower solar concentrations and it can work with organic devices and high band gap devices. Cadmium telluride has a very high band gap, so upconversion has the potential to add a bit to that, but the biggest impact will be in areas of high solar concentration.