Renewable energy is gaining ground. According to leading data and analytics company GlobalData, thermal power accounted for approximately 48% of global total capacity in 2024, with solar photovoltaic (PV) and wind making up 32%. However, by 2031 solar PV and wind are forecast to account for just over 50% of global total power capacity, indicating renewable’s increasing role in supplying baseload power. The expansion of renewables is the result of its increased cost-competitiveness with conventional energy sources, coupled with the growing urgency to decarbonise while also satiating increasing power demand.
What are the solutions to renewable intermittency and how can they ensure renewables reliably cater for baseload power?
Pumped storage hydropower (PSH) stands as a cornerstone of modern energy infrastructure, playing a vital role in balancing electricity supply and demand. As the only commercially proven storage technology at scale, it is the most widely used, accounting for 61% of total global energy storage capacity in 2024, according to GlobalData. Despite the prevalence of PSH, the popular answer today is increasingly battery energy storage systems (BESS). BESS is experiencing the largest capacity growth of any storage technology, with GlobalData forecasting a compound annual growth rate of 54% between 2020 and 2030. Its popularity is not unfounded, with BESS providing a flexible and modular energy storage system that can be deployed in conjunction with renewable energy systems, reducing the impact of their inherent intermittency. Although the cost of large-scale, long-duration BESS can be high, Li-ion solutions have become increasingly economically viable due to improvements in battery technology and the slower-than-anticipated adoption rate of EVs depressing lithium prices.
However, BESS comes with challenges. The use of large-scale lithium-ion battery systems increases safety concerns, including the risk of thermal runaway and fires. Thermal runaway occurs when the temperature of the battery begins to rise uncontrollably due to overcharging, excessive heat or mechanical damage. The higher temperature then causes faster charging, creating a positive feedback loop where the rate of chemical reactions inside the battery increases uncontrollably. These endless reactions often lead to the battery system catching fire or exploding, with the fire often being difficult to extinguish due to lithium-ion battery fires generating their own oxygen as well as releasing toxic gases, making it challenging to get to in proximity. For example, the Moss Landing lithium-ion battery energy storage plant in California, US, caught fire on 18 January 2025 – the fourth time since 2019. The fire destroyed 300MW of energy storage and forced the evacuation of up to 1,500 people. The reconstruction of the plant could take several years, and despite it only accounting for a 2% share of the state’s total energy storage capacity, the potential for reoccurrence or similar incidents at other battery plants poses a challenge to the transition towards renewables providing reliable generation. There were also concerns in California about the Los Angeles wildfires causing a chain reaction among lithium-ion battery facilities in the area. Moreover, rising global temperatures will increase the incidence of drought-induced wildfires, exacerbating the risk of battery fires. Authorities in Australia have labelled lithium-ion batteries as the ‘fastest growing fire risk’, with the New South Wales Fire and Rescue department reporting 272 responses to battery-related fires within the last year. These events present a cautionary tale when looking to scale BESS to balance renewable intermittency. Ensuring that manufacturing techniques and module separation are in line with safety regulations are essential to prevent and mitigate risks.
PSH can play a vital complementary role in the future of energy storage systems
Considering both the benefits and challenges of BESS, a balanced approach to energy storage is necessary, with PSH having the potential to be the optimal complementary solution. PSH is a mature technology, offering large-capacity, long life spans, and grid-friendly characteristics such as system balancing. This has resulted in renewed interest, with 477 PSH plants set to come online between 2025 and 2035, according to GlobalData. In 2024, China, Japan and the US were the leading countries utilising PSH, with approximately 55GW, 28GW, and 22GW of capacity, respectively. PSH also accounted for 95% of Japan’s total energy storage capacity in 2024.
PSH is a type of gravitational energy storage method, involving two reservoirs at different elevations. When electricity demand is high, water flows from the upper reservoir through penstocks to the lower reservoir, driving turbines to generate power. During low demand or surplus energy, water is pumped back to the upper reservoir. This process is a continuous cycle, ready to store or release energy, when necessary, by using stored gravitational energy. PSH can also be categorised as either open-loop or closed-loop. Open-loop involves the lower reservoir being connected to a continuous natural source of water while close-loop PSH systems are typically located off-stream. The latter is gaining popularity with a US Department of Energy report indicating that the environmental effects of closed-loop projects are generally lower than those of open-loop due to their location ‘off-stream’, minimising environmental impact and offering greater siting flexibility than open-loop projects.
The challenges of scaling PSH
The main challenge with PSH is the requirement for site-specific topographical conditions. However, companies are increasingly looking for solutions to increase its flexibility. The National Renewable Energy Laboratory authored a report that surveyed US landscapes for closed-loop PSH capacity and concluded there was still extensive potential, even when excluding areas of undevelopable land such as national parks and critical habitats for endangered species. Companies are also increasingly looking into closed-loop systems at disused quarries and pits. For example, the 250MW Kidston PSH scheme in Queensland, Australia, is being developed at an abandoned gold mine in conjunction with the 270MW K2S solar project. Another innovative project is RheEnergise constructing a 500KW demonstration project at a mining site near Plymouth, UK. The project uses a dense fluid called R-19, which enables the PSH system to be deployed on hills rather than mountains, providing equal power to traditional PSH projects but using 2.5 times less vertical elevation. If viable, the use of R-19 could reduce construction costs and time, a significant drawback to conventional PSH, while increasing the flexibility of PSH sites.
How well do you really know your competitors?
Access the most comprehensive Company Profiles on the market, powered by GlobalData. Save hours of research. Gain competitive edge.
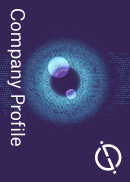
Thank you!
Your download email will arrive shortly
Not ready to buy yet? Download a free sample
We are confident about the unique quality of our Company Profiles. However, we want you to make the most beneficial decision for your business, so we offer a free sample that you can download by submitting the below form
By GlobalDataHowever, climate risk also impacts PSH through droughts, which has been evidenced by the experiences of hydropower-reliant countries. In late 2024, the Paraguay River hit record lows in Paraguay’s capital Asuncion, because of water levels being depleted by a severe drought upriver in Brazil. Similarly, in Zambia, an El Niño-induced drought had profound effects on the country’s water supply. Zambia and Paraguay are reliant on hydro for 88% and 100% of generation, respectively. As a result, both countries were subjected to prolonged blackouts due to a fall in generation. The arid conditions across both regions make attempts to scale PSH difficult, as its ability to provide grid stability will be significantly hindered during dry seasons.
The optimal solution for future energy storage systems
By 2030, GlobalData forecasts BESS to have a total global capacity of 1,200GW while PSH will have a capacity of 243GW. Both BESS and PSH have crucial roles to play in the future energy landscape. Despite BESS’ mineral resource dependency alongside persistent safety concerns around scaling BESS, it offers unmatched flexibility and scalability and can be deployed with renewable energies, making it vital to modern grid systems. Meanwhile, PSH offers unmatched capacity and lifespan, but is limited by geographic and environmental constraints coupled with complex and high-cost construction. As a result, PSH capacity will struggle to meet the burgeoning global demand for energy storage. To cement renewable energy’s role within the power mix, a diversified approach to energy storage is required. PSH and BESS should be utilised in conjunction with each other in an integrated approach to create a more flexible energy storage system that tackles intermittency issues, enabling a more reliable energy supply that supports the transition to a green energy grid.
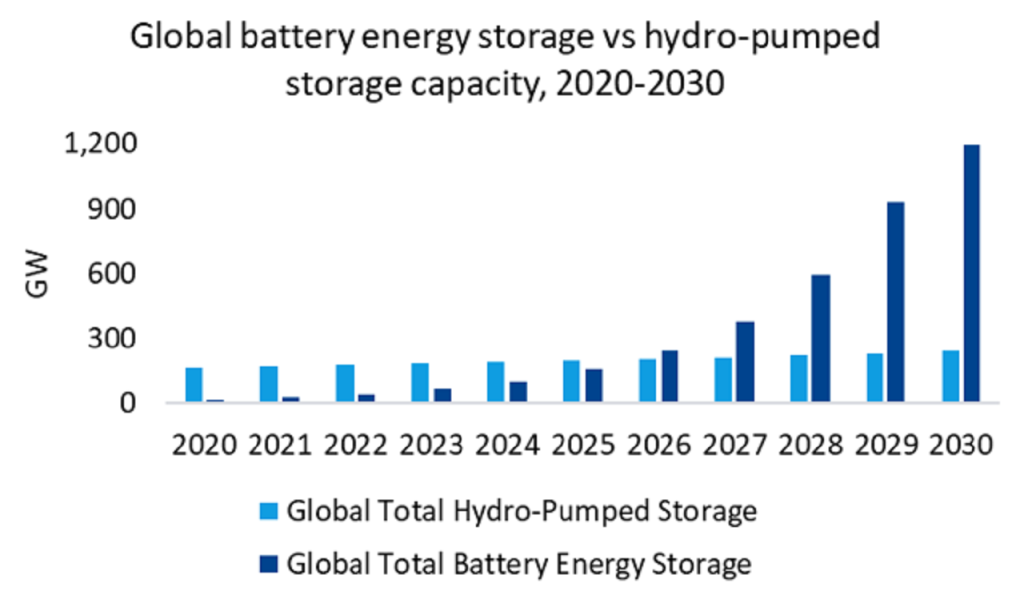