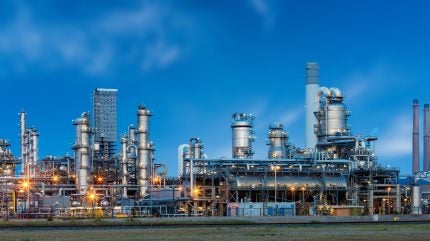
As 2024 marked the warmest year on record, the pressure is on to rapidly reduce global emissions, with much of the attention falling on the carbon capture, utilisation and storage (CCUS) industry.
Since the genesis of CCUS almost 50 years ago, carbon capture rates are now hovering at around 90–95% efficiency. The technology has become a central point of discussion within the global energy transition, as per the International Energy Agency (IEA)’s Net Zero by 2050 Scenario.
However, the associated economic and environmental costs mean that the technology also has its detractors. The Institute for Energy Economics and Financial Analysis (IEEFA) argues that the “long history of under-achievement” in CCUS only serves to perpetuate emissions.
As such, the improvement of capture rates is a critical mission for the industry. Traditional combustion methods are currently favoured to capture concentrated carbon dioxide (CO₂) at relatively low operating costs. However, emerging solutions such as direct air capture (DAC) are gaining ground based on the limited amount of land required and the removal of legacy emissions.
Streamlining the research and development (R&D) to commercialisation pipeline is crucial to improve carbon capture rates and consolidate the position of CCUS in the global energy transition.
Where do carbon capture rates currently stand?
Speaking to Power Technology, University of California, Berkeley professor of chemistry Omar Yaghi sets the scene for the challenge facing the CCUS industry. “At present, there are approximately 1,100 gigatons of excess CO₂ in the atmosphere released due to the burning of fossil fuels. This is the CO₂ we need to remove from the air.”
Carbon capture is typically used in large point sources and heavy industrial processes such as power plants, oil and gas refineries and cement production, the latter of which is solely responsible for 8% of global CO₂ emissions.
For small-scale operations, such as sites emitting up to 100,000 tonnes of CO₂ annually, the IEA Greenhouse Gas Programme points out that there is a “scarcity of data” on the impact of carbon capture. However, across different sectors and scales, 90% continues to be the baseline rate.
According to Massachusetts Institute of Technology’s (MIT) TILclimate podcast, 90% efficiency is targeted for its technical achievability and financial practicality. “To catch the last remainders of CO₂ once a system passes 90% it is an equal parts engineering puzzle and economics problem,” says Howard Herzog, senior research engineer at the MIT Energy Initiative.
The percentage is established through a multi-step process of measurement, reporting and verification (MRV). A baseline is used to measure abatement against and net removals are reported through national inventory reports under the UN Framework Convention on Climate Change and Paris Agreement or registries to the Greenhouse Gas Protocol.
Following a comparison of measured and predicted performance of the carbon capture through adsorption, an independent third-party verifies the removal.
Research from the International Journal of Greenhouse Gas Control finds that “in the context of a 1.5°C target, going beyond 90% capture will be vital”. However, the “economically viable deployment” of CCUS is a significant barrier to increased efficiency.
The costs and benefits
As with small-scale implementation, there is a scarcity of detailed and accurate information on the costs of MRV, but the London School of Economics (LSE) estimates that it accounts for 5% of total CCUS investment, with the rest going towards equipment and energy usage.
Francesca Gregory, senior energy transition analyst at Power Technology’s parent company, GlobalData, explains that “the energy intensity of removing the remaining CO₂ (as you are working against the concentration gradient) makes it prohibitively expensive and so it is a cost-benefit trade-off”.
Prateek Bumb, co-founder and chief technology officer of carbon capture solutions provider Carbon Clean, is confident that capture rates can “considerably exceed” 90–95% but concurs that “cost has long been a barrier to adoption. Both the initial outlay and the operating costs required for large conventional plants with high columns are hard to justify for many emitters, particularly small-to-mid-size facilities.”
Carbon Clean addresses total installed costs by shrinking the size of carbon capture units based on a modular design, exemplifying the influx of emerging solutions that continue to progress CCUS.
Key mature and emerging technologies
Combustion solutions are the bedrock of point-source carbon capture, with three methods offering variations in efficiency and energy consumption depending on project requirements.
Post-combustion removes CO₂ after burning fossil fuels, capturing it from flue gases at low pressure using amine solvents, sorbents or membranes. This is the most mature method and can be retrofitted to existing emissions sources for staged implementation – but significant energy is used in the separation process.
In pre-combustion, concentrated CO₂ is captured at a higher pressure using either chemical or physical absorbents before fossil fuels are burned. This method accounts for 75% of global active CCUS capacity and is most used within natural gas processing and gasification power plants.
The most nascent form of combustion capture is oxyfuel, which reduces the temperature of oxygen flue gas to remove the bulk of the nitrogen content from the air before combustion occurs. This results in lower energy requirements than post-combustion due to the easier separation of water vapour and CO₂, resulting in an almost pure concentration.
Post-combustion and oxyfuel combustion have both enabled 95% capture rates while pre-combustion lags behind at 80–90%, although success varies between sectors. For example, post-combustion is a well-proven and commercially available solution in the petroleum and coal-fired power industries, but the world’s first carbon capture plant in the cement industry only achieved mechanical completion in December 2024.
The main alternative to combustion is DAC, an atmospheric solution that is expected to grab more than 980 million tonnes of CO₂ towards the 2050 net zero target.
DAC separates CO₂ from ambient air using solids or liquids. This allows for deployment at a wide range of locations and the reduction of residual emissions, which are a byproduct of combustion capture methods.
However, CO₂ captured from the atmosphere is more dilute than from a point source. This requires high temperatures and energy intensity to re-release the CO₂ for industrial use and regenerate the solvents or sorbents for another capture cycle.
The IEA confirms that emerging DAC technologies rely on innovative separation systems and chemical compositions. The University of California, Berkeley recently made major progress in this area by developing a new absorbing material.
As the inventor of covalent organic frameworks (COFs), Yaghi explains that the latest version, COF-999, is “special for its porosity, stability, versatility and ability to selectively adsorb CO₂ over other gases, adding to its significance in carbon capture.
“We know that COFs have the potential to be an exceptional carbon capture material because of their superior properties, and we have been working hard to chemically modify them. Compared to other pre and post-combustion capture methods, COF-999 stands out for its potential to reduce costs and energy consumption while achieving high capture rates.”
In the broader CCUS industry, the time to market for innovations is typically slow. Yaghi has worked for 20 years on strengthening COFs, with each amine able to capture approximately one CO₂ molecule, and he is bullish about the technology’s commercial potential.
“The synthetic route for COF-999 is relatively straightforward, making it accessible for research, industrial applications and scaling up for commercial use in DAC within two years,” he says. Speed and efficiency are of the utmost importance for carbon capture solutions, as Gregory confirms that “active CCUS capacity currently minimally contributes to progress towards emission targets”.
Capturing carbon for a net-zero future
GlobalData forecasts that the current dominance of pre-combustion capture will shift towards post-combustion at 62% of active capacity by 2030, led by increased adoption across power generation and heavy industry facilities.
Oxyfuel combustion and DAC are also expected to increase in active capacity but continue to contribute minority shares to the overall CCUS landscape. This balance could tip if emerging solutions are successfully commercialised at scale.
“The journey from lab to commercialisation requires an unrelenting focus on testing, optimisation and validation,” says Bumb. “We are focused on making carbon capture as replicable as electric vehicle batteries and solar panels. Repeatability is essential so that plants can be rolled out quickly and at the necessary scale to reduce global emissions.”
Digitalisation is another developing avenue, as Bumb explains that AI can be used for targeted predictive maintenance in carbon capture plants. “It bridges the gap between theoretical ideal operating conditions and the reality of practical deployment, such as variations in temperature.”
CCUS will continue to be a contentious topic unless carbon capture makes a significant impact on global emissions. Indeed, at COP29, the number of industry lobbyists in attendance exceeded those of key delegations, sparking further accusations of enabling fossil fuel activity.
Despite this, Gregory is optimistic about progress in CCUS. “The technology is increasingly being recognised for its potential role in the decarbonisation of existing, heavy-emitting industrial assets,” she says. “Countries such as the UK see the technology as critical for reaching net zero by 2050.”
Looking at the broader purpose of carbon capture, Yaghi emphasises that technological development isn’t the only piece of the puzzle. “Now, the responsibility lies with society to take the next step – to commit to addressing this pressing issue and introduce a cost for emitting carbon, urging us toward a more sustainable and conscious future.”